EPR [wiki] is a magnetic resonance technique which detects the resonance transitions between energy states of unpaired electrons in an applied magnetic field. The electron has spin, which gives it a magnetic moment. The magnetic moment makes the electron behave like a tiny bar magnet. When we apply an external magnetic field, the paramagnetic electrons can either orient in a direction parallel or antiparallel to the direction of the magnetic field. This creates two states with different energies ±½gµBB0 . g is the g-factor, which is a proportionality constant equal to 2.0023 for free electron, but strongly dependent on electronic envionment of the species. µB is the Bohr magneton [wiki], which is the natural unit of electronic magnetic moment. The splitting of the energy levels by applied magnetic field is called the Zeeman effect [wiki]. The difference between the spin state energies can be probed by application of the additional magnetic field oscillating with the frequency ν, such that ΔE = hν. Here ΔE is energy difference between levels and h is Planck constant. This field will drive the electron between the states only if the resonance condition ΔE = hν = gµBB0 is satisfied.
What is Electron Paramagnetic Resonance?
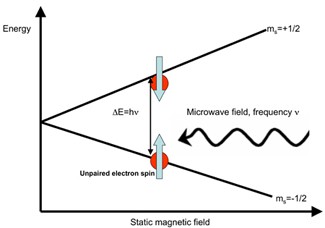